Western United States Upper-mantle Anomalies - Dynamic Modeling Project
WestUS Home | People | Dangling Slab | Lithospheric Drip | Publications
Dangling Slab Dynamics
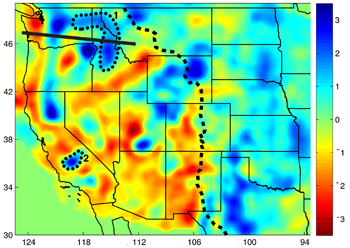
Fig. 1 Bird's eye view of structures below the Western U.S. - This horizontal tomographic section shows the structure of the upper mantle at 195 km depth. The color indicates the relative speed of the seismic waves when passing through a region. Red means the wave slows down, indicating the material there has low density. Blue means the wave speeds up, indicating the material has high density. The linear, high-density (blue) feature extending from northern California through Oregon and Washington is the "Gorda-Juan de Fuca" slab of the active Cascades subduction zone. The two labeled areas are structures of unknown origin, and thus areas of current research. The thick black line running through feature #1 is used to indicate the cross section in Figure 2. Modified from: Schmandt and Humphreys, 2010a
|
|
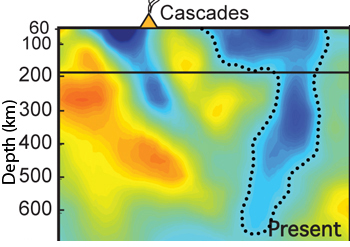
Fig. 2 Cross section of dangling curtain - indicated by dotted line. See Fig. 1 caption for explanation of color scale. Modified from: Schmandt and Humphreys, 2010a
|
Recent seismic imaging of the mantle beneath the western United States reveals complexities interpreted to be structures ranging from plumes to drips and dangling fragments of subducted plates.
Our research focuses on one such feature, lying beneath northern Idaho and western Washington (labeled #1 in Figure 1). This feature hangs suspended in the upper mantle much like the curved fold of a curtain. Figure 2 shows a side view of this dangling "curtain" (profile taken along the heavy black line in Figure 1).
Some questions we'd like to answer are
- Where did this feature come from? Is it a piece of previously subducted plate?
- And if it is a dangling remnant of a plate, why is it still hanging there?
There has already been some work in this area by other groups. For example, based on tomography and geological observations in the region (which indicate the tectonic and volcanic history of the area), Schmandt and Humphreys (2011) proposed a conceptual model that interprets this feature to be a stalled remnant of the subducted Farallon plate. This interpretation is shown in Figure 3:
- 60 Million years ago (60 Ma), the subduction zone had a kink in it (Figure 3, first panels of rows A and B).
- Ten Million years later (50 Ma), the subduction at the kink "stalled" and re-initiated farther to the west, to be more in line with the rest of the subduction zone (second panels of rows A and B in Figure 3, 50 Ma).
- The remnant slab was left dangling in the upper mantle, while a new subducted slab was forming nearby (third panels rows A and B in Figure 3, 40 Ma).
- Whereas the dangling slab may be expected to have detached and sunk deeper into the mantle, in this model the slab remains dangling for the last 40 My to the present configuration shown by the tomographic image on the far-right panel of Figure 3B.
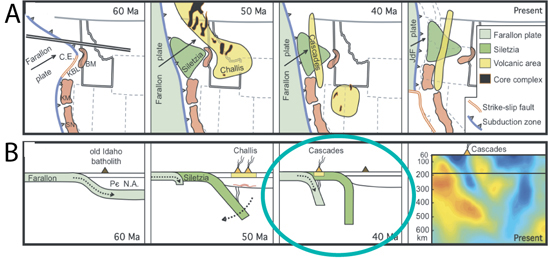
Fig. 3 Current interpretation of the sequence of events leading to the formation of the dangling curtain - Upper panels provide a bird's eye view of the volcanic history and geological observations. Lower panels provide a cross-sectional view of the proposed sequence of events in cartoon form, as well as a current-day tomographic section, taken along the solid line in Figure 1. The blue circled frame represents the time (40 Ma) and geometric scenario from which we start our model. In this interpretation, the slab has been dangling since this time. From: Schmandt and Humphreys, 2010a
|
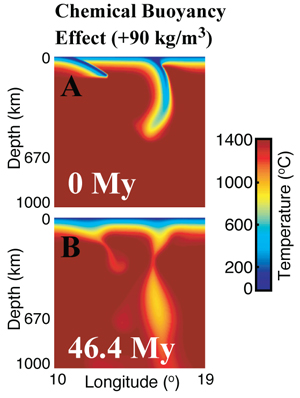
Fig. 4 Case without subduction and with additional 90 kg/m3 slab chemical buoyancy - (A) Initial position of dangling slab (same for all model runs), time = 0 My. (B) Position of dangling slab at time of detachment, 46.4 My, for the case of additional chemical buoyancy.
|
If this dangling curtain is truly a remnant of the subducted Farallon Plate, then the question we'd like to answer is
- What are the conditions under which a slab fragment might persist in the upper mantle (e.g., for at least 40 My) rather than rapidly detach and sink deeper within the earth’s interior?
We run a time-varying, two-dimensional numerical model, varying conditions in the model to find what lengthens the residence time of the slab. Some of the model inputs or conditions that we vary include
- rheology (how rock flows)
- time-dependent processes (e.g., active subduction)
- density (affects buoyancy)
Our model uses a version of the mantle convection code CitcomS [Zhong et al. (2000); Tan et al. (2006); and see Burkett and Billen (2009) for viscosity law and parameters specific to these models].
We start our dynamic models with the scenario represented by the circled frame in Fig. 3. Initial conditions, shown in Figure 4A, define a subducted plate that stalled when the slab was 500 km deep, referred to as the stalled or ‘dangling’ slab (feature to far right), as well as a re-initiated subduction zone shifted 450 km west of the ceased subduction zone (shallower feature on the left). As a control, we also run the model without subduction of the westward plate.
Model results:
- Case A: No nearby subduction - For our reference case, we start out with a 500 km deep dangling slab, no added chemical buoyancy, and no active nearby subduction. We find that detachment occurs within 16 My, which is much earlier than the >40 My residence time predicted by the Schmandt et al. (2011) interpretation shown in Figure 3.
- Case B: No nearby subduction but increased chemical buoyancy - To see whether the slab can be made to dangle longer (again without nearby subduction), we added additional buoyancy to the slab (offsetting the thermal negative buoyancy with a positive chemical buoyancy). We found that we needed to add 90 kg/m3 to increase the residence time to the required 40 - 50 My range (Figure 4B). However, this slab vs. mantle density contrast (~3%) is unrealistic. Thus chemical buoyancy alone cannot reasonably explain such long dangling times.
-
Case C: No nearby subduction, but increased lower-mantle viscosity - Greater lower-mantle viscosities provide more viscous support of a sinking slab and thus causes sinking velocities to slow down. We ran the model with a range of lower-mantle viscosities, from 1.4 times that of the upper-mantle viscosity to 100 times that of the upper mantle viscosity. We found that the time it took for the slab to detach increased with increasing lower-mantle viscosity:
Lower-mantle viscosity increased by a factor of: | Resulting slab residence time (My): |
1.4 | 18 |
10 | 20.4 |
30 | 21.8 |
100 | 22.3 |
For the case of a two-order-of-magnitude viscosity increase, slab detachment was delayed by about 5 My.
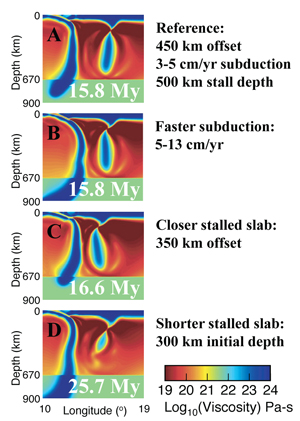
Fig. 5 Model results for cases with nearby subduction
A) Case D (Nearby subduction is slow) - Slight delay seen in slab detachment, with noticeable tilt of detached slab toward the neighboring subduction zone
B) Case E (Same as Case D but nearby subduction is faster) - Still little effect seen on the time of detachment, but detached slab is seen to be even closer to the subduction zone
C) Case F (Same as Case D but slab initially placed closer to subduction zone) - Detachment is delayed by about 1 My, and the nearby shallow part of the subducting plate is seen to flatten out a bit, in response to a suction effect in the small wedge between the two slabs
D) Case G (Same as Case D but initial slab is shorter, just 300 km in depth) - the time to detachment is lengthened to 25.6 My, and the stalled slab it deflected markedly toward the nearby subduction zone
Colors indicate viscosity for full model domain.
|
-
Case D: Nearby subduction - We found that when active subduction is nearby, only a minor change (delay) of < 0.5 My results. The more notable effect is a slight westward deflection of the stalled slab toward the active subduction zone (see Figure 5A).
-
Case E: Nearby subduction, faster rate - When we increase the rate of the nearby subduction from ~3-5 cm/yr (as in Case D), to ~5-13 cm/yr, there is no change in the time the slab takes to detach. However, there is a stronger geometric effect in both the dangling slab and the subducting slab. The dangling slab deflects further toward the nearby subducting slab, and the subducting slab shows an even greater deflection toward the dangling slab, as well as a flattening out of its shallow end (compare Figure 5B with 5A).
-
Case F: Nearby subduction, closer slab proximity - Starting the dangling slab off at 350 km, rather than 450 km, from the nearby subducting slab, causes detachment to be delayed by ~1 My. The shallow part of the nearby subducting plate flattens out in response to suction created in the even smaller region between the two slabs (as seen in Figure 5C).
-
Case G: Nearby subduction, shorter stalled slab – When we start with a stalled slab that is shorter (with a depth of only 300 km), detachment occurs later, at 25.6 My. Thus detachment for the shorter dangling slab takes ~10 My longer than for the heavier, 500 km deep slab. Also, the shorter dangling slab is deflected even more notably toward the subduction zone (as seen in Figure 5D).
-
Case H: Nearby subduction, with initial conditions chosen to obtain the longest delay to detachment – For this case we chose initial conditions (all within reasonable ranges) that delay detachment (e.g., 100x jump in lower mantle viscosity, short stalled slab at 300 km depth, 15 kg/m^3 chemical density, no nearby subduction). The resulting detachment time was only 28.3 My. While this was the longest time of any of our physically reasonable model runs, it is not long enough to explain the proposed residence time of 40 - 50 My for the dangling slab beneath the northwestern US.
Conclusion - These cases demonstrate the possibility of dangling slab remnants lingering in the upper mantle for nearing 30 My (Case H), largely based on combining the delay effect of a reasonable additional chemical buoyancy and a 300 km deep initial slab. The presence of nearby active subduction has nearly negligible effect on the timing of the stalled slab’s detachment, but does notably lead to westward deflection of the stalled slab, which is consistent with the observed geometry (Figure 2). The dangling times in these models do not however reach the proposed residence time of up to 40-50 My as for the inferred dangling remnant slab beneath the northwestern US. Therefore, future models will need to further constrain the dynamic, rheologic, and geometric (particularly in 3-D) conditions that might allow for dangling slab remnants to linger even longer in the upper mantle.
Contacts: Erin Burkett and Mike Gurnis
|